Kīlauea Lava Flow Continues Dramatic Ocean Entry
Kīlauea continues to erupt at its summit and East Rift Zone. This past week, the summit lava lake level raised in concert with summit inflation, from a low of about 52 m (171 ft) to as high as 12 m (39 ft) below the vent rim within Halema‘uma‘u Crater. The 61g lava flow continued to enter the ocean near Kamokuna. The lava flow does not pose an immediate threat to nearby communities.
Mauna Loa is not erupting. Seismicity remains elevated relative to the long-term background rate, with small earthquakes occurring mostly in the volcano’s south caldera and upper Southwest Rift Zone at depths less than 5 km (3 mi). Global Positioning System (GPS) measurements show deformation related to inflation of a magma reservoir beneath the summit and upper Southwest Rift Zone.
Two earthquakes were felt on the Island of Hawai`i in the past week. On Friday, October 7, 2016, at 2:21 p.m., HST, a magnitude-3.2 earthquake occurred 7.4 miles (12 km) southeast of Honoka’a at a depth of 5.6 miles (9 km). On Sunday October 9, 2016, at 3:44 a.m., HST, a magnitude-3.9 earthquake occurred 9.3 miles (15 km) east of Leilani Estates at a depth of 8.1 miles (13.1 km).
As a strong caution to visitors viewing the 61g flow ocean entry (where lava meets the sea), there are additional significant hazards besides walking on uneven surfaces and around unstable, extremely steep sea cliffs. Venturing too close to an ocean entry exposes you to flying debris created by the explosive interaction between lava and water. Also, the new land created is unstable because it is built on unconsolidated lava fragments and sand. This loose material can easily be eroded away by surf, causing the new land to become unsupported and slide into the sea. In several instances, such collapses, once started, have also incorporated parts of the older sea cliff.
Finally, the interaction of lava with the ocean creates a corrosive seawater plume laden with hydrochloric acid and fine volcanic particles that can irritate the skin, eyes, and lungs.
2006 Kīholo Bay earthquakes spur monitoring improvements:
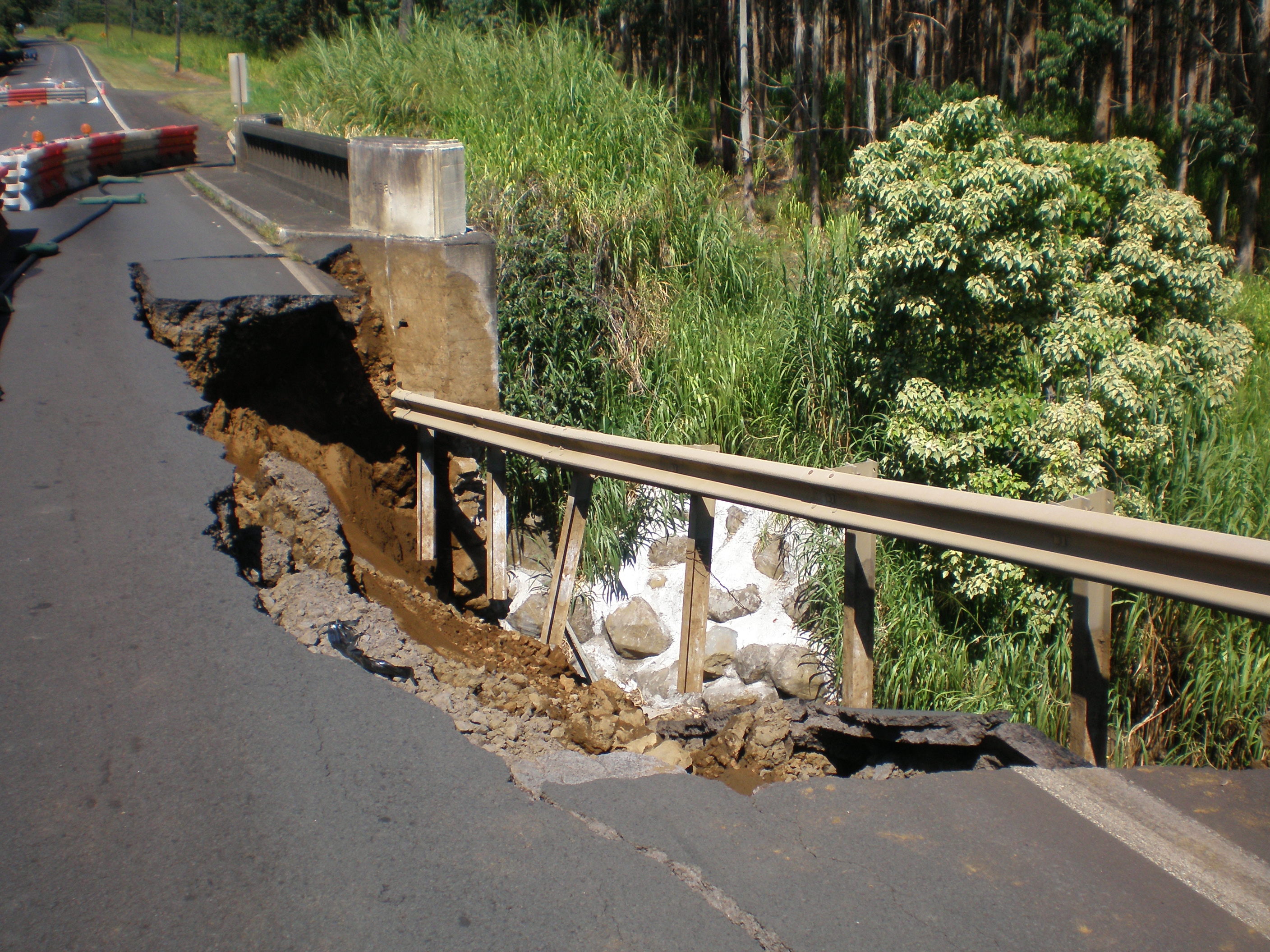
Damage along Hawaii Belt Road at Kawaili Bridge, near Paauilo, following the Kīholo Bay and Māhukona earthquakes of October 15, 2006. USGS photo.
Ten years ago shortly after 7:00 a.m., HST, on Sunday, October 15, 2006, two damaging earthquakes struck off the northwest coast of the Island of Hawai‘i—a magnitude-6.7 earthquake beneath Kīholo Bay and a magnitude-6.0 earthquake offshore of Māhukona.
The earthquakes damaged roads and buildings and impacted utilities in the Kohala, Kona, and Hāmākua Districts of Hawai`i County and on the eastern side of Maui. O‘ahu experienced a major power outage. The impacts might have been far worse if the earthquakes had struck on a weekday.
When USGS and Pacific Tsunami Warning Center (PTWC) seismologists saw the signals from the Kīholo Bay earthquake, it was immediately clear the signals were much larger than the thousands of microearthquakes that occur each year. Their immediate questions were, “How large was the earthquake?” and “Would there be a damaging tsunami?”
In 2006, seismic monitoring across the United States was already computer-based; data were transmitted from remote field sites and collected at seismographic monitoring centers, and then converted into computer-ready formats. The USGS had initiated its Advanced National Seismic System (ANSS) project to modernize earthquake monitoring in the Nation. Older field instruments were gradually being replaced with units that streamed digital data—computer-ready signals—to regional and national earthquake processing centers.
At the time, three seismic stations on the Island of Hawai`i had been outfitted with digital instruments as part of an upgrade to improve U.S. tsunami warning capabilities. A handful of other seismic stations had already been upgraded with digital units as part of the initiative to resolve the Year 2000 Problem (Y2K). Although staff at the USGS’s Hawaiian Volcano Observatory (HVO) assisted with these upgrades, these units were not yet fully integrated with HVO’s volcano-monitoring network.
Within minutes of the Kīholo Bay earthquake, seismologists verified that it was deep, roughly 40km (~25miles). Although the earthquake shook HVO instruments beyond their operating range, data from the tsunami-upgraded instruments on the Island of Hawai`i yielded the first estimate of magnitude-6.6 as determined by PTWC. Several minutes later, after telephone calls among seismologists at PTWC, HVO, and the USGS’s National Earthquake Information Center in Colorado, a consensus was reached of magnitude-6.7. Based on earthquake depth and magnitude, PTWC confirmed that there was no tsunami threat from the earthquake.
The Y2K-upgraded stations were not equipped to transmit data in real-time, but they nevertheless provided important information about the earthquake. These instruments recorded even the strongest shaking of both earthquakes. With this data, USGS seismologists in Colorado created ShakeMaps to show how shaking from the earthquakes varied from place to place. Such information, along with the Kīholo Bay “Did You Feel It? Community Internet Intensity Map,” guided and informed post-earthquake surveys of potential damage and other effects as recovery efforts began.
A USGS assessment in the aftermath of the 2006 earthquakes recognized the need to integrate more modern digital instruments and analysis systems into HVO’s earthquake monitoring program. For example, in order analyze in real time data from the new digital field instruments, a new ANSS Quake Management Software (AQMS) system developed and used in California was needed at HVO (installed in 2009).
Subsequently, with support from the American Reinvestment and Recovery Act (ARRA) of 2009, HVO modernized all seismic field instruments and radio systems. Simply put, HVO now records all earthquakes of interest beneath the Island of Hawai‘i with the means to automatically generate state-of-the-art earthquake information products. Important work continues to maintain the instruments and radio systems, and also to improve the automated and rapid delivery of reliable earthquake information.
These improvements will help in the analysis of future large-magnitude earthquakes and in understanding and anticipating their impacts.
The 10 years since the Kīholo Bay and Māhukona earthquakes have brought many new residents to Hawaii’s earthquake country. Most of our elementary school students probably have no direct recollections of the 2006 earthquakes. Next week’s annual Great Hawaii ShakeOut drill to practice Drop! Cover! and Hold On! is a reminder to everyone about Hawaii’s earthquake history and how to prepare for and recover from future earthquakes.
The Great Hawaii ShakeOut takes place at 10:00 a.m., HST, on Thursday October 20, 2016. For more detailed information about earthquake preparedness, please visit the Great Hawaii ShakeOut website (http://shakeout.org/hawaii/).
Kamakaiʻa Hills: what are they and why are they there?
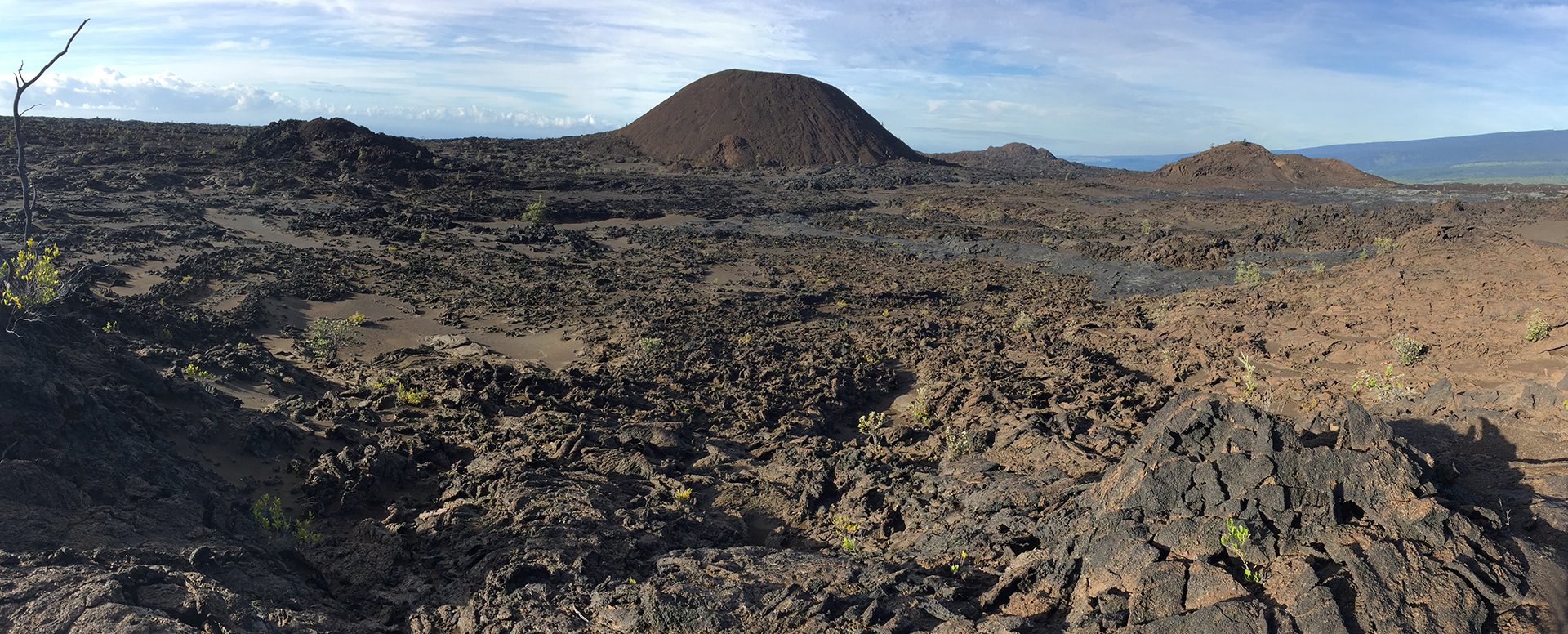
Shown here are three of the main volcanic cones (center and right) that make up the Kamakaiʻa Hills on Kīlauea Volcano’s Southwest Rift Zone. Findings from a study of the Kamakaiʻa Hills that is currently underway by the USGS Hawaiian Volcano Observatory will likely revise the known geologic history of this remote area on Kīlauea and provide new insights on past and future eruptions along the volcano’s Southwest Rift Zone. USGS photo.
Visitors to the Jaggar Museum and Kaʻū Desert in Hawaiʻi Volcanoes National Park, struck by the appearance of three dark, symmetrical volcanic cones on the western slope of Kīlauea Volcano, often ask “what are they?” and “why are they there?”
The cones are the Kamakaiʻa Hills. Their Hawaiian name means “the eye of the fish,” possibly because the cones, each dimpled with a cup-shaped crater, reminded early Hawaiians of the eyes on prized fish, such as ulua.
The Kamakaiʻa cones are merely the largest of a series of vents, including older, more eroded cones, spatter ramparts, and large ground cracks, that spewed lava clots and blocks of older rock for short periods. Multiple eruptions have originated along this three-mile long “mini-rift” over a period spanning at least 500 years.
The two largest eruptions, each probably lasting weeks to a few months, produced far-travelling flows and rootless lava shields similar to those that have grown around Kīlauea’s East Rift Zone Pu‘u ‘Ō‘ō vent over the last three decades. The big Kamakaiʻa cones developed during explosive phases of the eruptions, producing fields of volcanic bombs, rubbly scoria, and spatter. The largest bombs in these ejecta beds exceed 1 m (3 ft) in diameter.
Unusual eruption products at the Kamakaiʻa Hills correspond with a form of lava that has no equivalent elsewhere on Kīlauea—pasty pāhoehoe with a distinctively stretched “skin” that resembles the grain one might find on pieces of old driftwood. This lava is also chemically distinctive.
Preliminary analyses of the lava indicate that at least some of it contains much more silica than ordinary Kīlauea basalt. In fact, it is similar to basaltic andesite, a type of lava abundant in the Coast Range of Oregon and northern California. This suggests that the magma beneath the Kamakaiʻa Hills was stored for a long time before it erupted to the surface, which allowed it to evolve to a greater degree than lava found anywhere else on Kīlauea. Such chemical evolution might also explain its explosiveness.
Initial efforts to establish the ages of the Kamakaiʻa Hills lava were based on palemagnetism—measurements of ancient orientations of Earth’s magnetic field preserved in the basalt. That study concluded that the Kamakaiʻa flows were older than the Footprints Ash from the A.D.1790 explosive eruption, which marked the onset of historically recorded eruptions at Kīlauea.
However, current investigations of the Kamakaiʻa Hills show that two flows are younger than the 1790 Footprints Ash. These flows probably erupted sometime between 1790 and 1823, when the first Euro-Americans visited the volcano. Reverend William Ellis, leader of that first expedition, commented that he observed “smoking chasms” in the vicinity of the Kamakaiʻa cones—highly suggestive of recent volcanic activity there.
Geologists also recently discovered sets of fossil human footprints in the Footprints Ash deposit within the Kamakaiʻa Hills. This significantly extends the area in which people are known to have been moving immediately following the 1790 eruption.
To explain why the Kamakaiʻa Hills exist requires combining several critical strands of research, including geophysics, structural geology, and geochemistry. Findings from a study presently underway at the USGS Hawaiian Volcano Observatory will likely revise the known geologic history of Kīlauea’s Southwest Rift Zone.
The Kamakaiʻa Hills are positioned where the Koaʻe Fault System, which National Park visitors can easily view along the Hilina Pali Road, merges with Kīlauea’s Southwest Rift Zone. They terminate westward at a bend in the rift zone near Puʻukou, a site of ongoing shallow earthquake activity.
Seismicity over the past few decades suggests that magma periodically intrudes from Kīlauea’s summit reservoir southward to the Koaʻe Fault System, then bends to follow the Southwest Rift Zone into the area beneath the Kamakaiʻa Hills. Puʻukou could act as a “log jam,” causing long-term storage of the trapped magma beneath the Hills.
Repeated eruptions in the Kamakaiʻa Hills might occur because occasional intrusions of fresh magma drive the older, more evolved magma to the surface. Continuous southward sliding of Kīlauea’s seaward slope might also keep the Kamakaiʻa Hills corridor open and volcanically active.
Past events provide important insights into Kīlauea’s future. Knowing this, we certainly expect that eruptions of unusual character are likely to break out again in this remote and interesting area on the volcano.
How big is that earthquake? Why magnitudes sometimes change
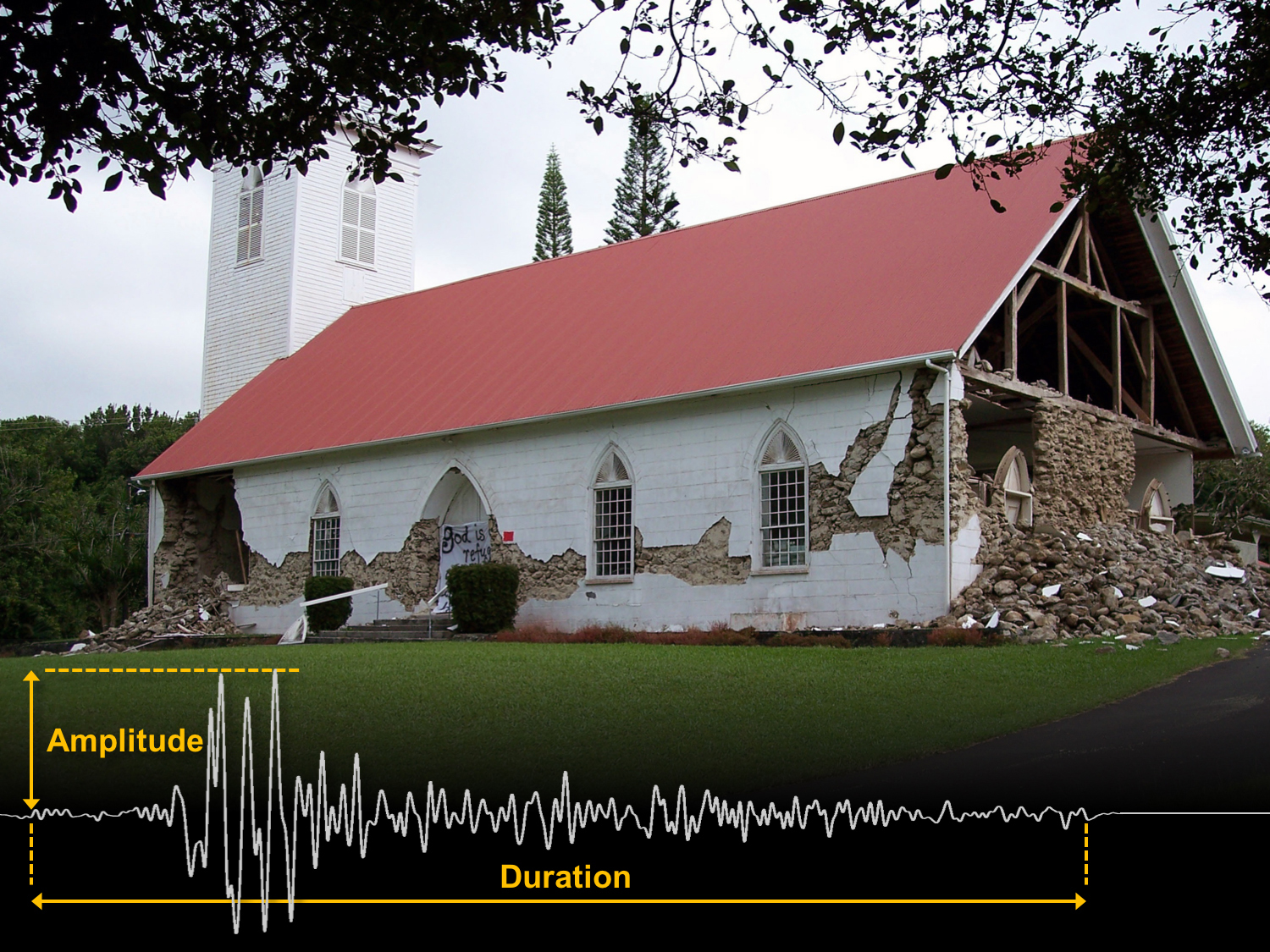
The Kalāhikiola Congregational Church in Kapa‘au was extensively damaged on October 15, 2006, by two earthquakes (magnitudes 6.7 and 6.0) off the northwest coast of Hawaiʻi Island. The earthquakes were felt throughout the State of Hawaii, but the greatest damage occurred in the North Kona and Kohala Districts of the Island of Hawaiʻi. An example of a seismogram (bottom of photo) illustrates the relation between the amplitude and duration of shaking, which is used by seismologists to compute earthquake magnitudes. USGS photo.
Characterizing earthquakes is one of the most important activities we do at the USGS Hawaiian Volcano Observatory (HVO). Seismicity helps us monitor the “pulse” of volcanoes and can be a first indication of an impending eruption.
Earthquakes also pose a hazard in their own right, and the entire State of Hawaii is at risk from damaging earthquakes. They occur abruptly, so we must be vigilant and prepared.
HVO posts preliminary information about earthquake location and size (magnitude) as soon as possible after one occurs. Later, we update the information as more data becomes available. Sometimes the reported magnitude changes during this process.
When the earth shakes, vibrating seismic waves radiate outward from the source. These waves are picked up by sensitive instruments called seismometers, which are located across the state. Seismic data is relayed in near real-time to HVO, where computers trained to look for earthquake patterns keep watch around the clock. When four or more stations detect an earthquake, the computer automatically estimates the location and magnitude of the event.
If the earthquake magnitude is above 3.0, the computer posts the information to HVO’s website (http://hvo.wr.usgs.gov) without human intervention. The USGS Earthquake Notification Service (https://sslearthquake.usgs.gov/ens/) then alerts subscribers. This generally happens within five minutes of the earthquake.
HVO’s seismologists then spring to action to review the data. They recalculate the earthquake parameters and, if needed, overwrite the previously posted automatic ones. For magnitude-4.0 and higher earthquakes, we complete our manual review within two hours and issue a news release. Events smaller than magnitude-4.0 are reviewed routinely within a few days.
Upon review by a seismologist, the earthquake’s magnitude can go up or down by a few tenths. Different groups, such as the Pacific Tsunami Warning Center and the USGS National Earthquake Information Center, might also report slightly different magnitudes. To understand these differences, let’s review what magnitude means.
The concept of earthquake magnitude dates back to 1935, when Charles Richter created a way to compare the relative sizes of earthquakes in southern California. He measured how the amplitude of shaking recorded by seismometers decreased with distance from an earthquake. Using the logarithm of the maximum amplitude, Richter was able to derive a scale that conveyed the wide range of earthquake sizes, which can vary by several orders of magnitude (hence the name). For example, on this scale, the amplitude of a magnitude-4.0 earthquake is ten times higher than that of a magnitude-3.0 earthquake.
Today, there are numerous types of earthquake magnitudes that take advantage of advances in seismic instrumentation and cover a wide range of situations. For earthquakes higher than about magnitude-5.5, the most common measure of magnitude is called moment magnitude, which relates fundamentally to the energy released by an earthquake’s fault motion. For every 0.2 increase in moment magnitude, the energy doubles. For example, a magnitude-6.2 earthquake releases roughly twice as much energy as a magnitude-6.0 event.
For smaller earthquakes, like those that happen daily in Hawaii, HVO computes two types of magnitude based on either the duration or amplitude of the shaking recoded by seismometers. Duration magnitude tends to work better for smaller earthquakes (less than about magnitude-2.0) that are located shallower than 20 km (12 mi) beneath the surface. Amplitude magnitude, which is a modern-day formulation of Richter’s magnitude, works better for local earthquakes with magnitudes between about 2.0 and 5.5.
Any reported magnitude is actually an average of values computed for each seismometer that recorded the earthquake. These values vary depending on distance, direction, instrument, and the type of material type along the seismic wave’s path. HVO seismologists evaluate which stations provide the most reliable and objective magnitude estimates, average them together, and select either duration or amplitude as the preferred magnitude type for each event.
Magnitudes can change following a seismologist’s review and analysis of the computer’s automatic magnitude assignment. This process provides the best information available at any given time and offers a window into how the science of real-time seismology unfolds.
What matters more than a number, however, is what you do if you feel shaking during an earthquake. On October 20, we encourage you to take part in the world’s largest earthquake preparedness exercise by practicing “Drop, Cover, and Hold On!” during the Great Hawai‘i ShakeOut (http://shakeout.org/hawaii).
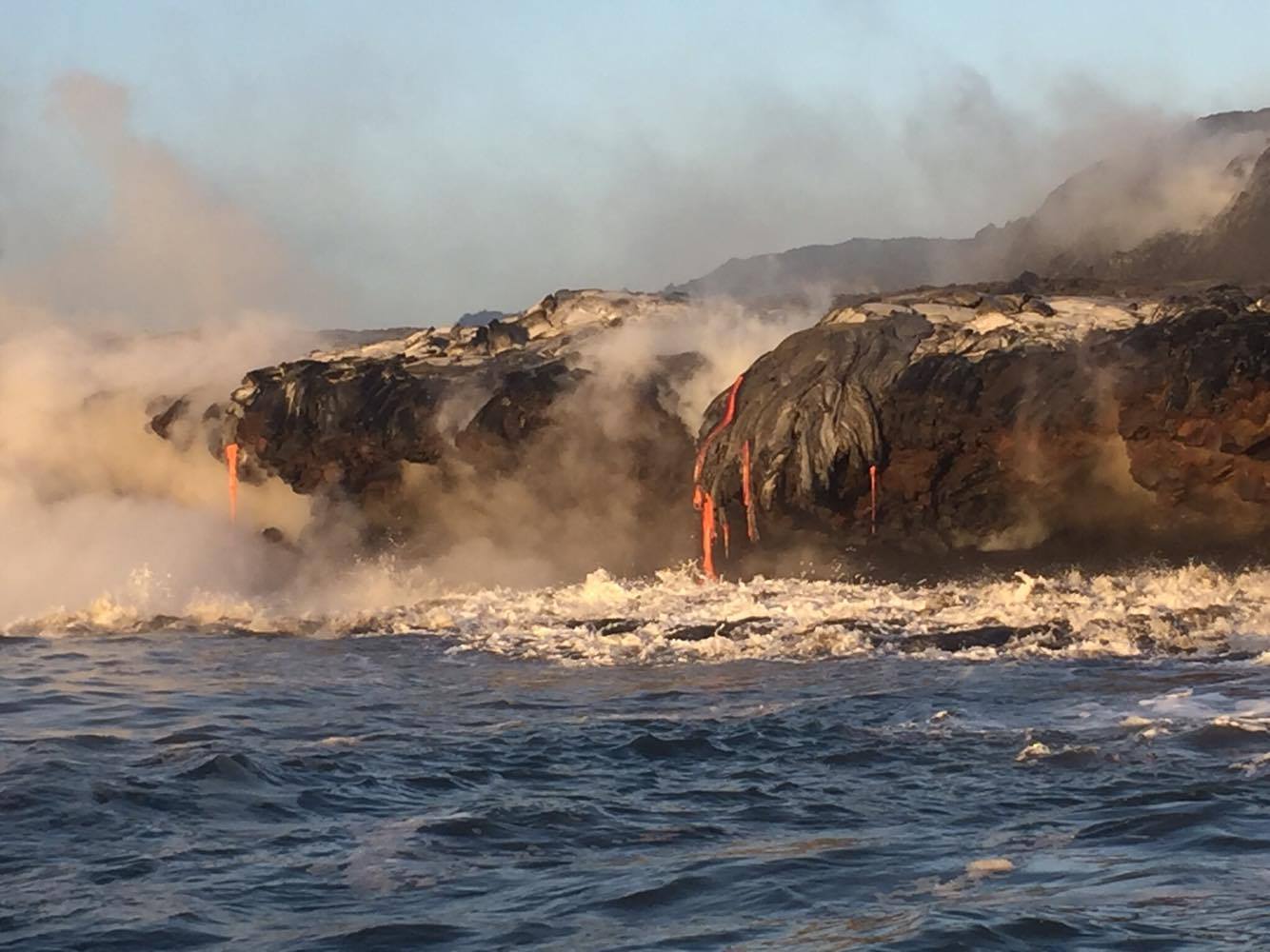
Kīlauea lava ocean entry, 10.13.16. PC: Robin Pilus.
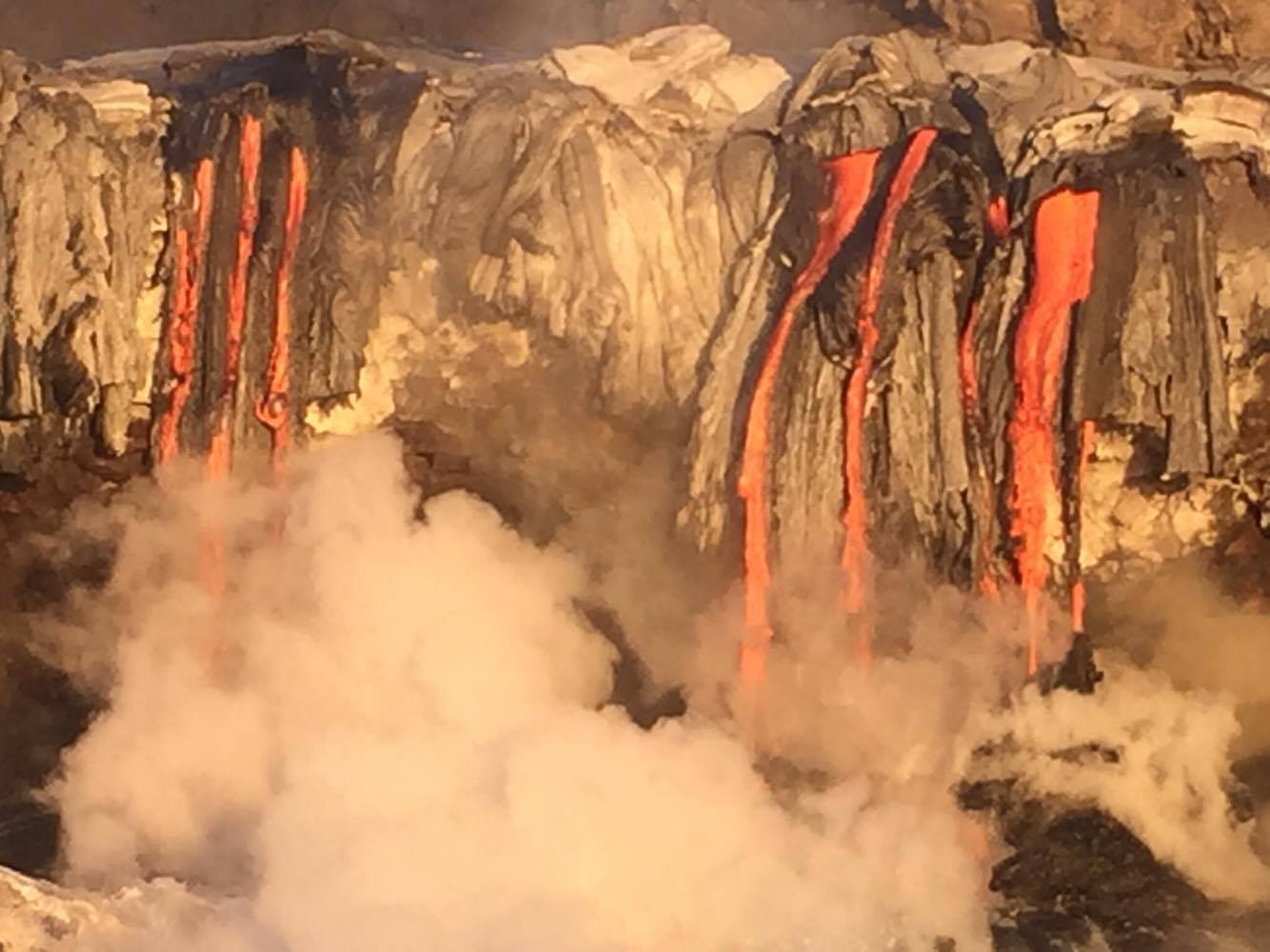
Kīlauea lava ocean entry, 10.13.16. PC: Robin Pilus, special thanks to Captain Skosh – Hoʻokupu Lava Tours/ http://bigislandlavaboat.com/.
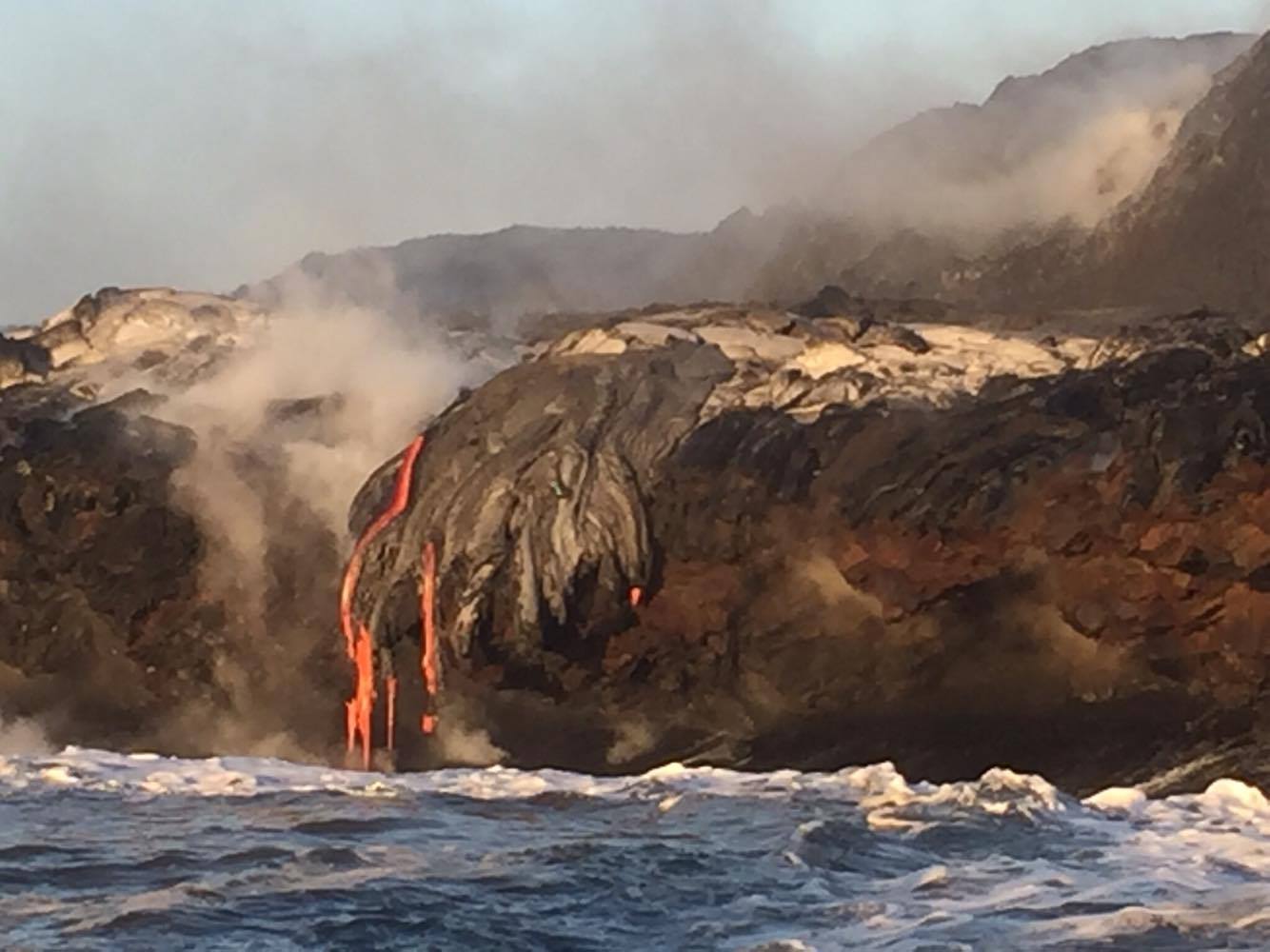
Kīlauea lava ocean entry, 10.13.16. PC: Robin Pilus.
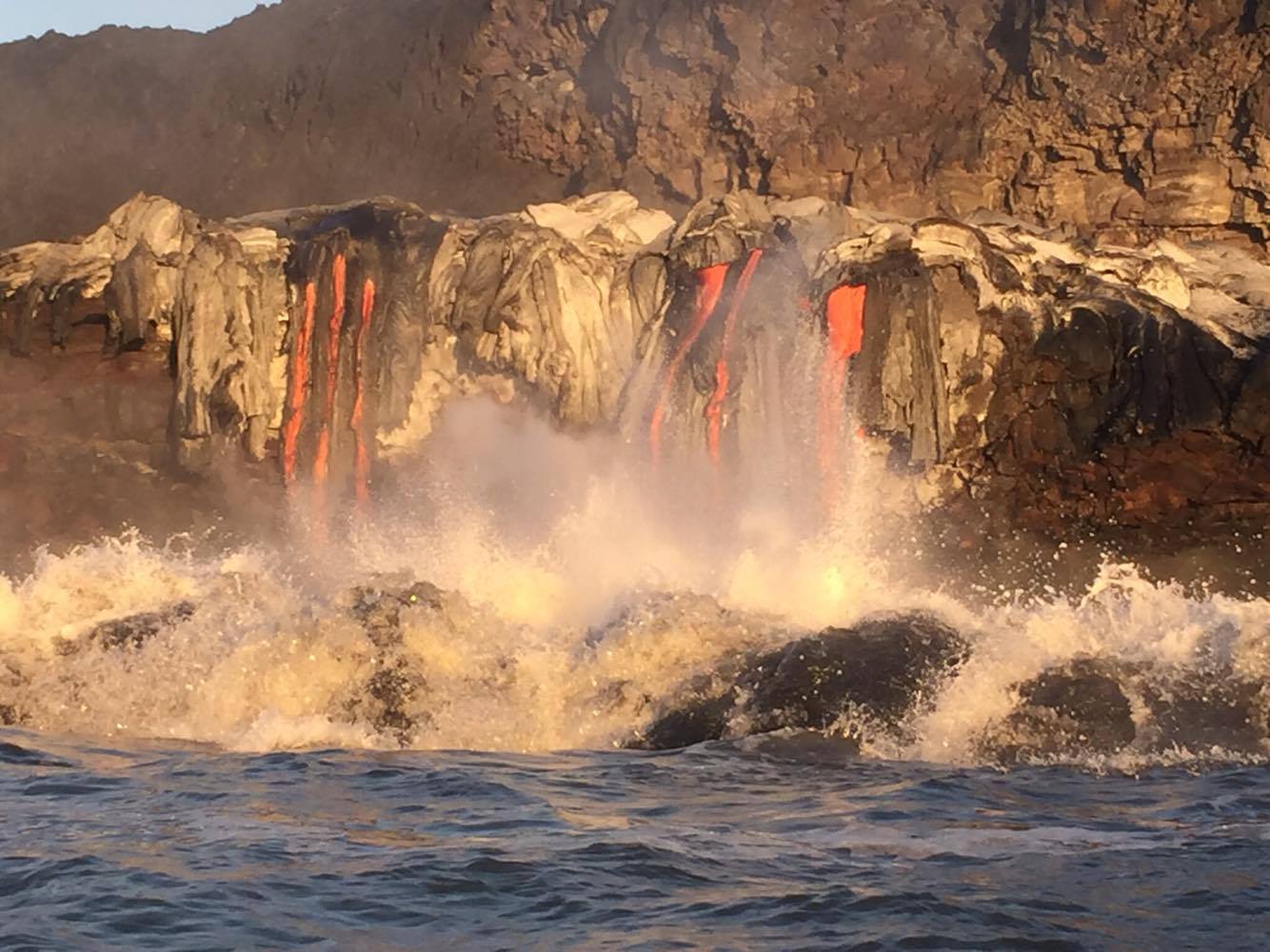
Kīlauea lava ocean entry, 10.13.16. PC: Robin Pilus.
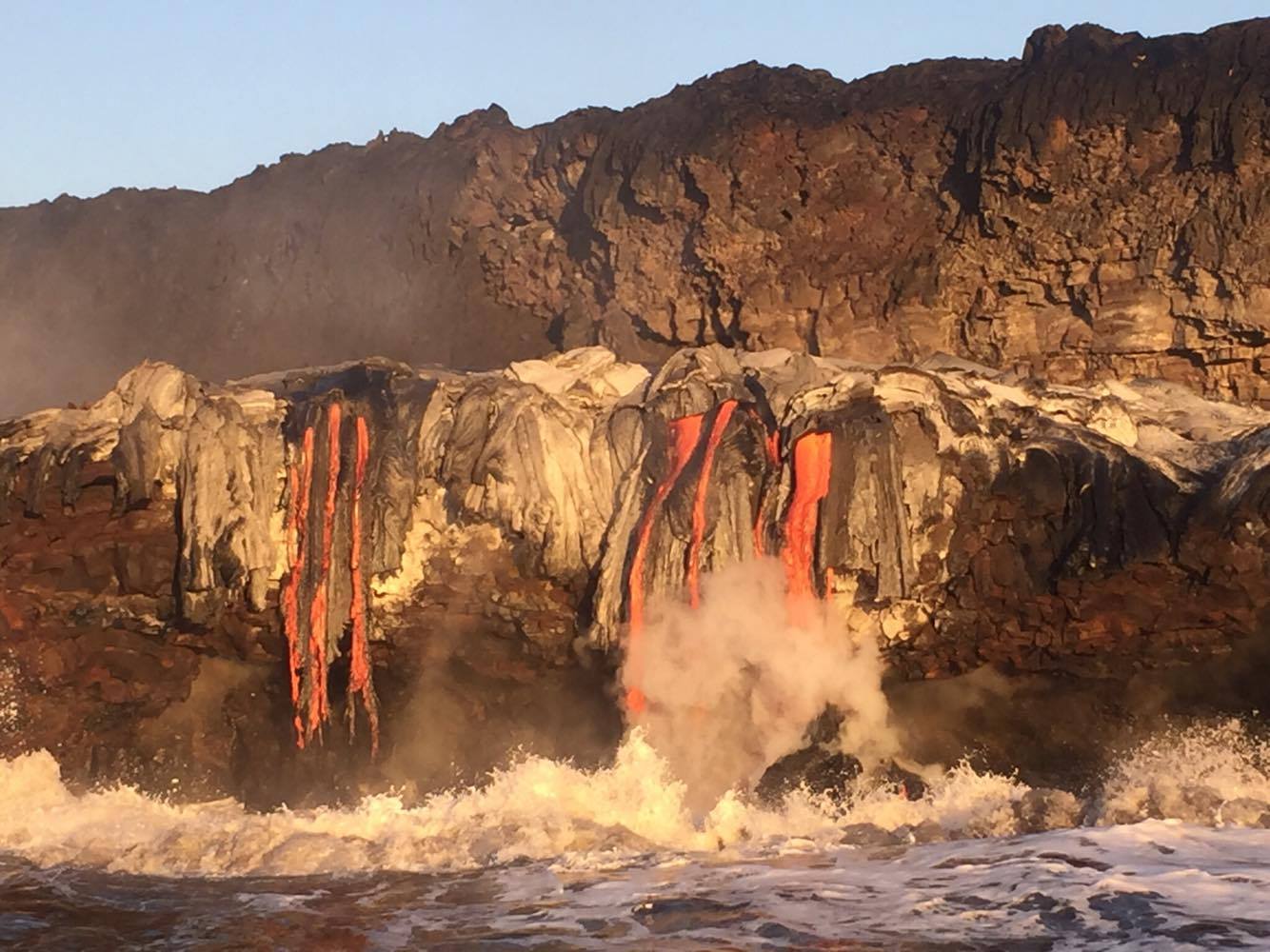
Kīlauea lava ocean entry, 10.13.16. PC: Robin Pilus.
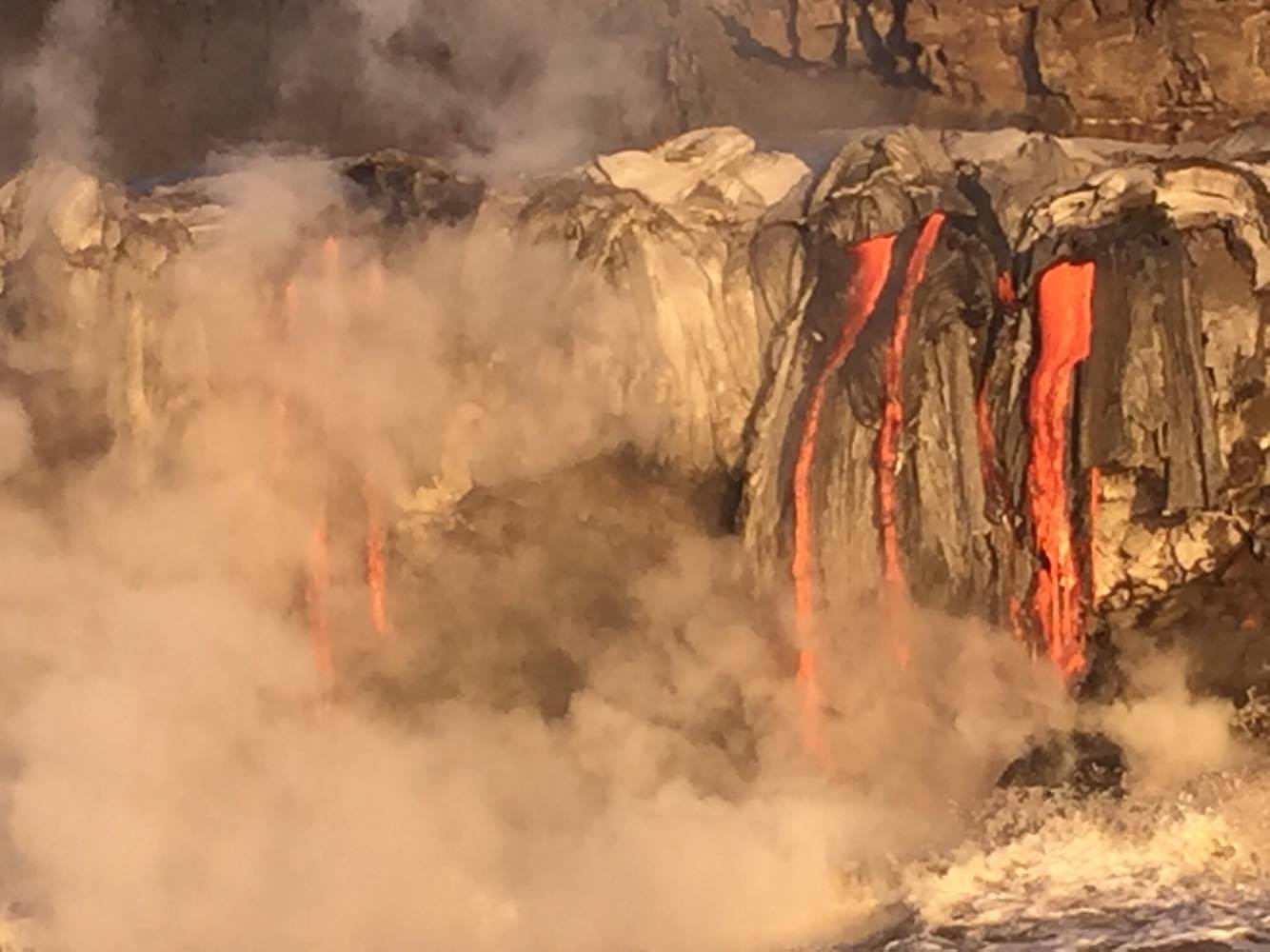
Kīlauea lava ocean entry, 10.13.16. PC: Robin Pilus.
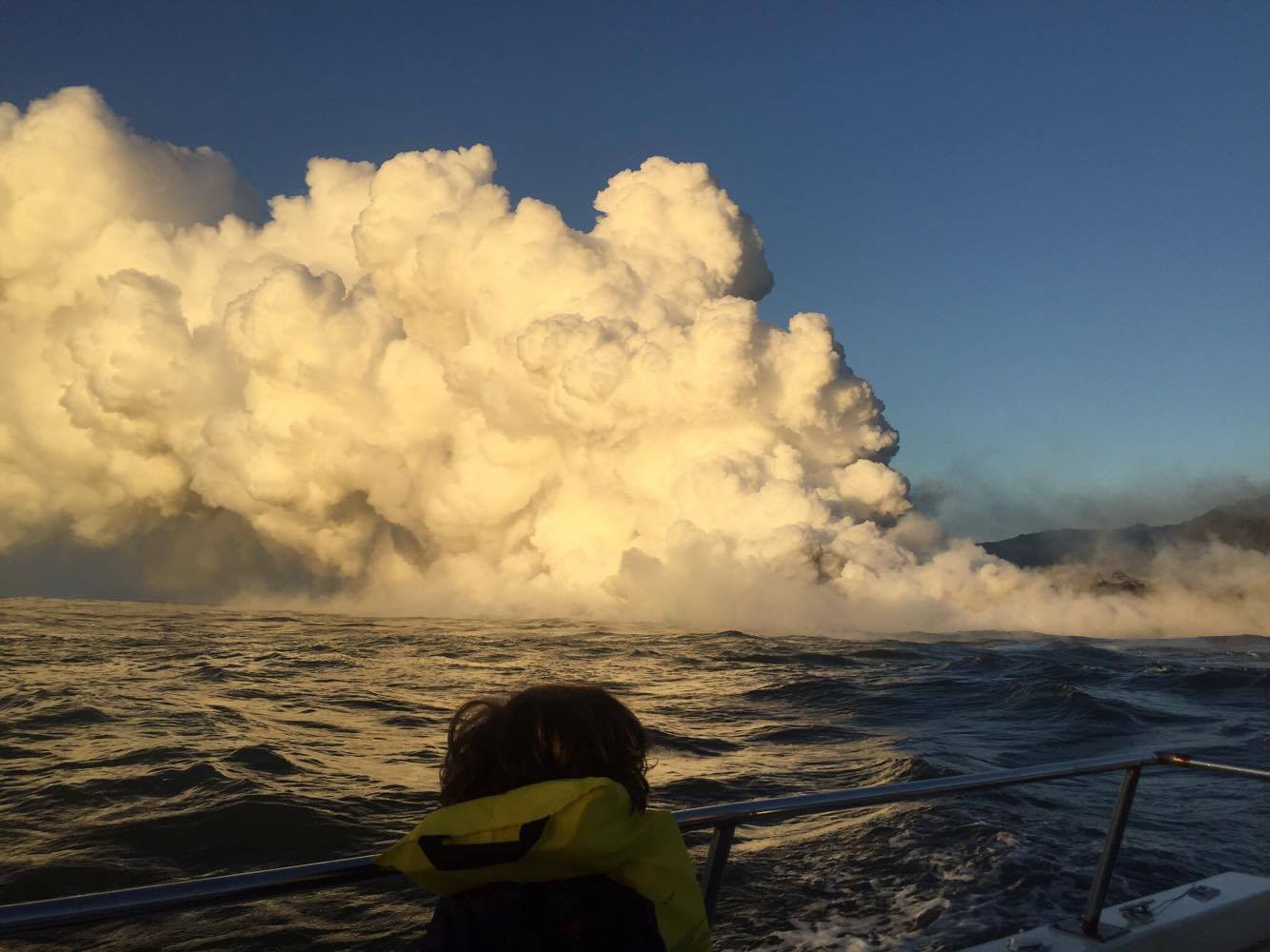
Kīlauea lava ocean entry, 10.13.16. PC: Robin Pilus.
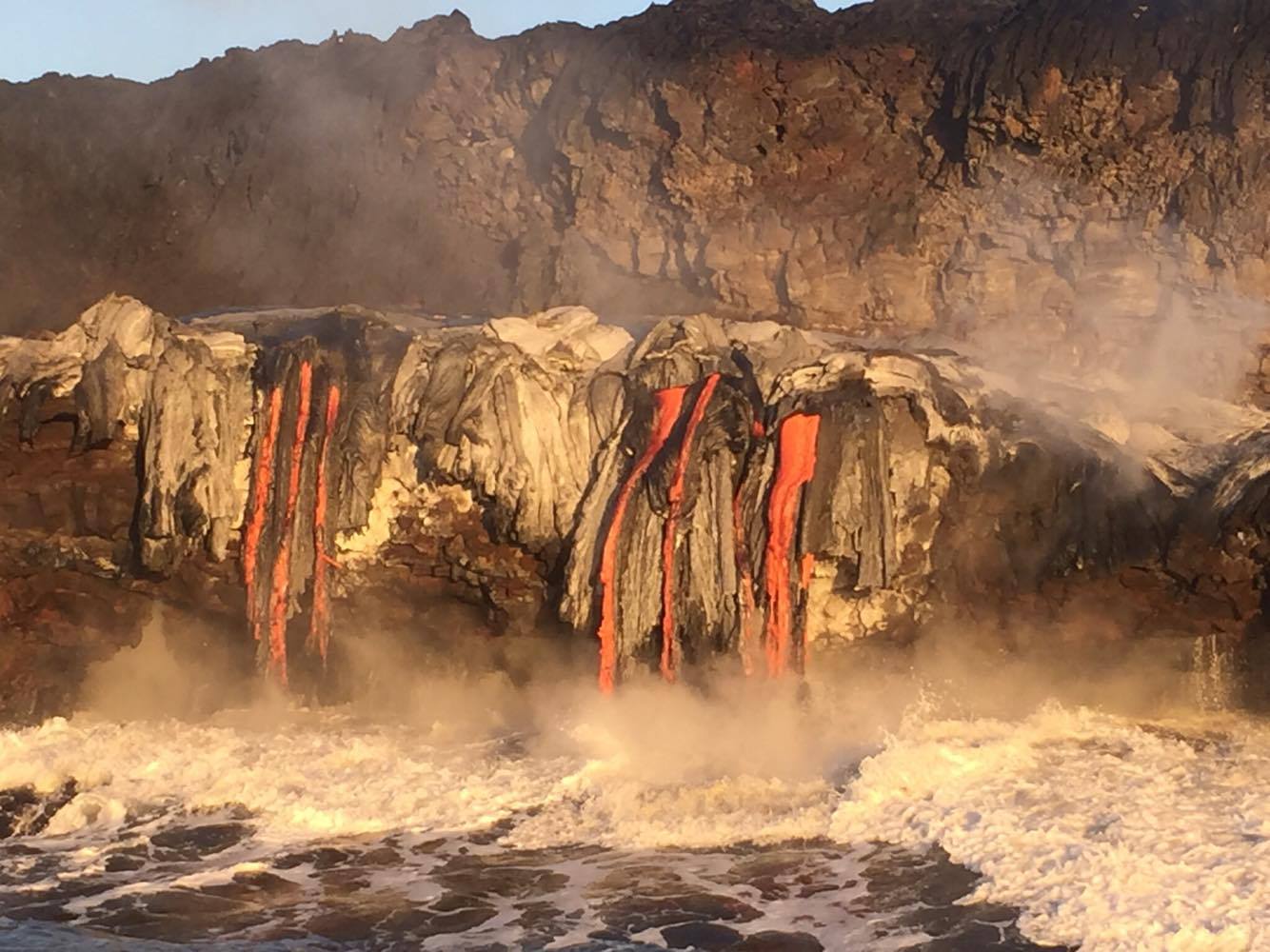
Kīlauea lava ocean entry, 10.13.16. PC: Robin Pilus.
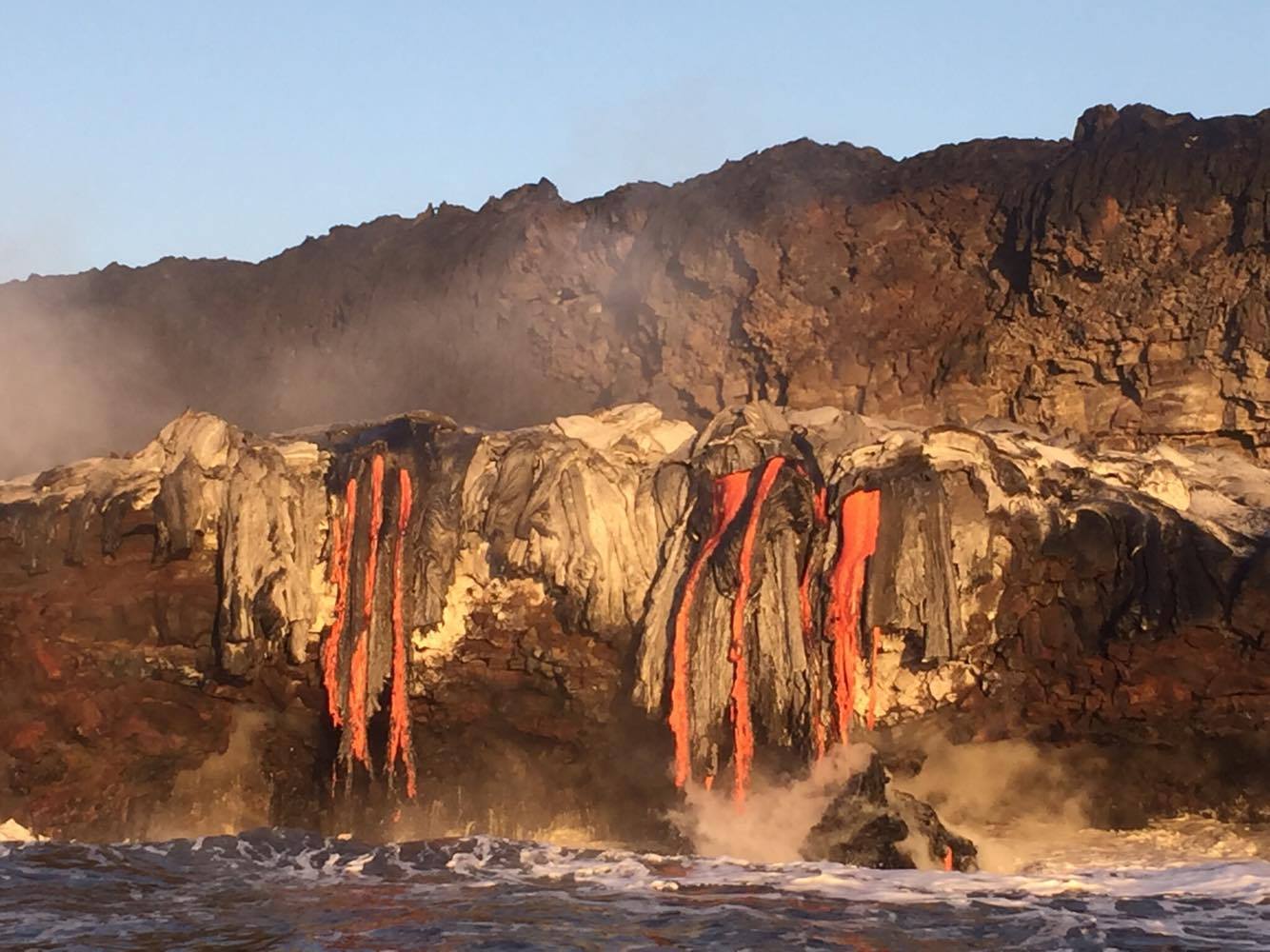
Kīlauea lava ocean entry, 10.13.16. PC: Robin Pilus.
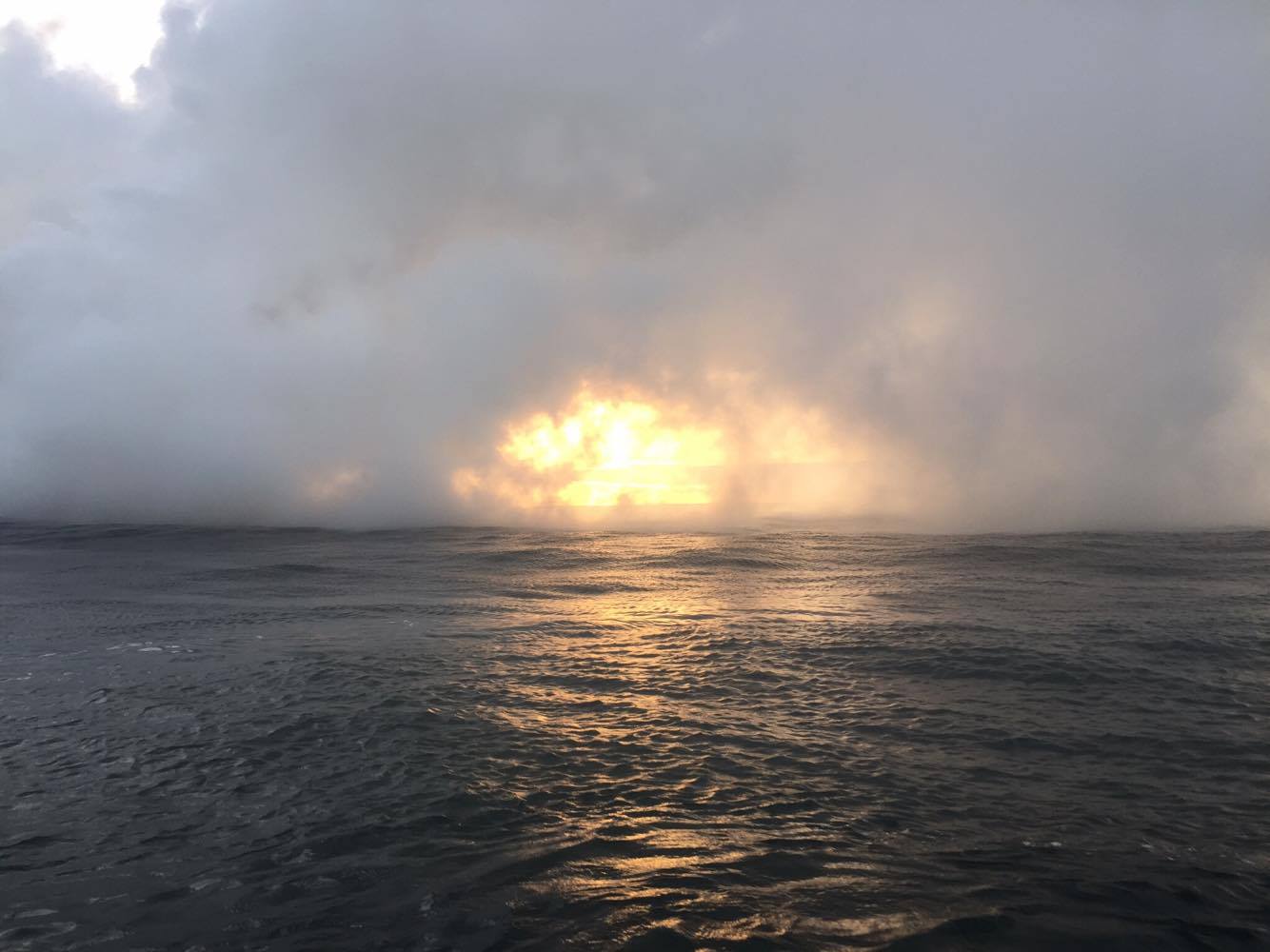
Kīlauea lava ocean entry, 10.13.16. PC: Robin Pilus.
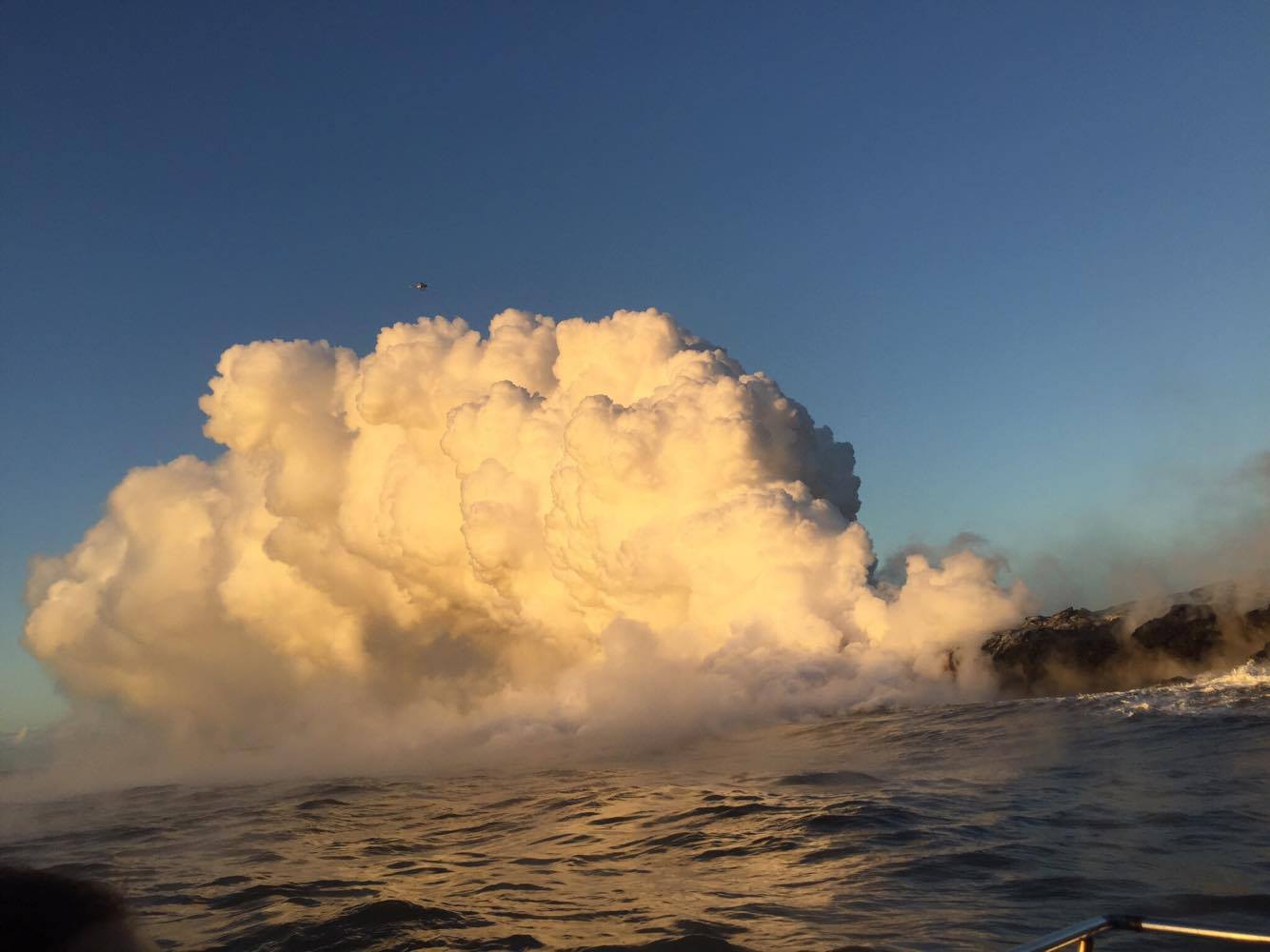
Kīlauea lava ocean entry, 10.13.16. PC: Robin Pilus.
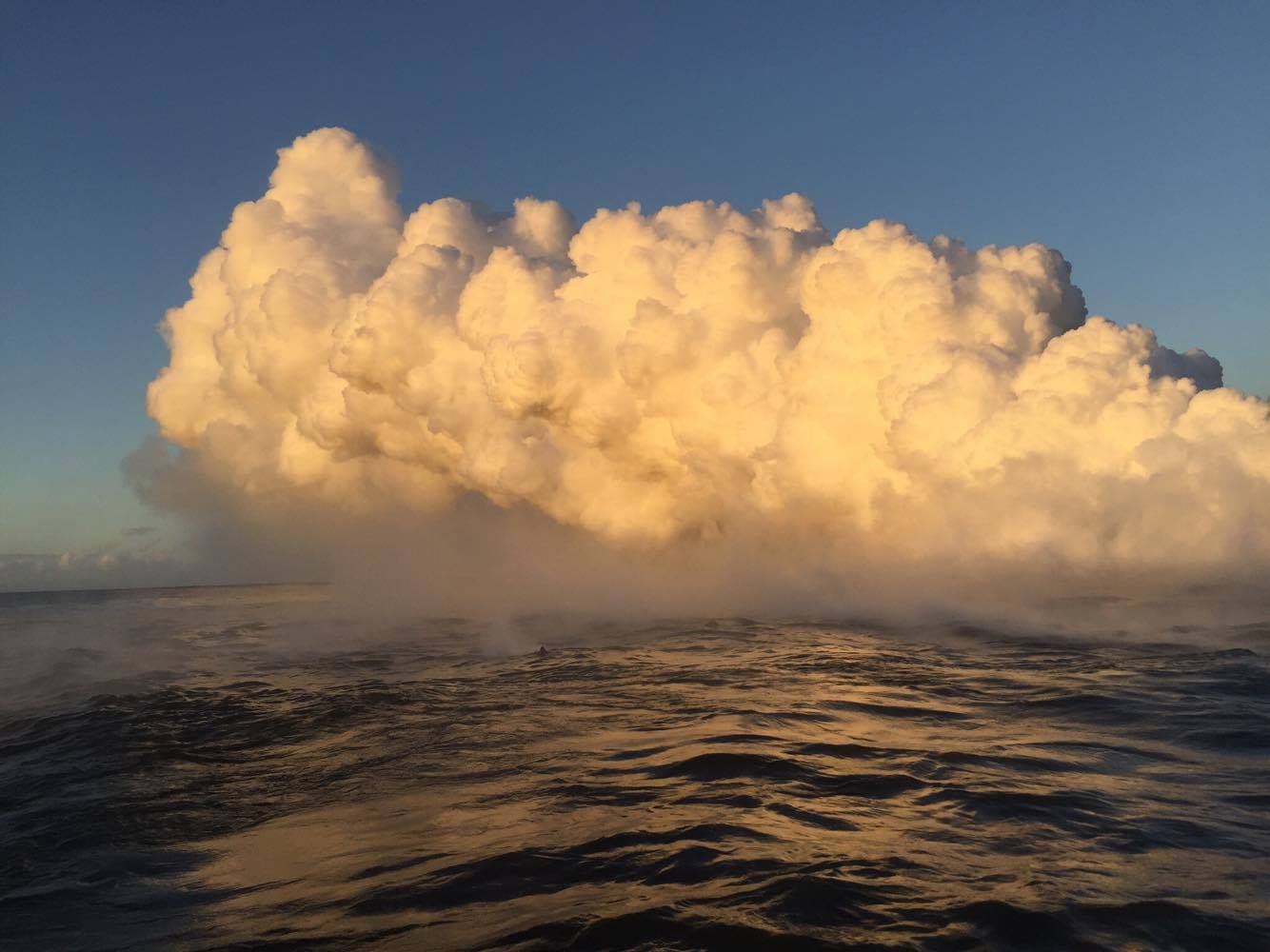
Kīlauea lava ocean entry, 10.13.16. PC: Robin Pilus.
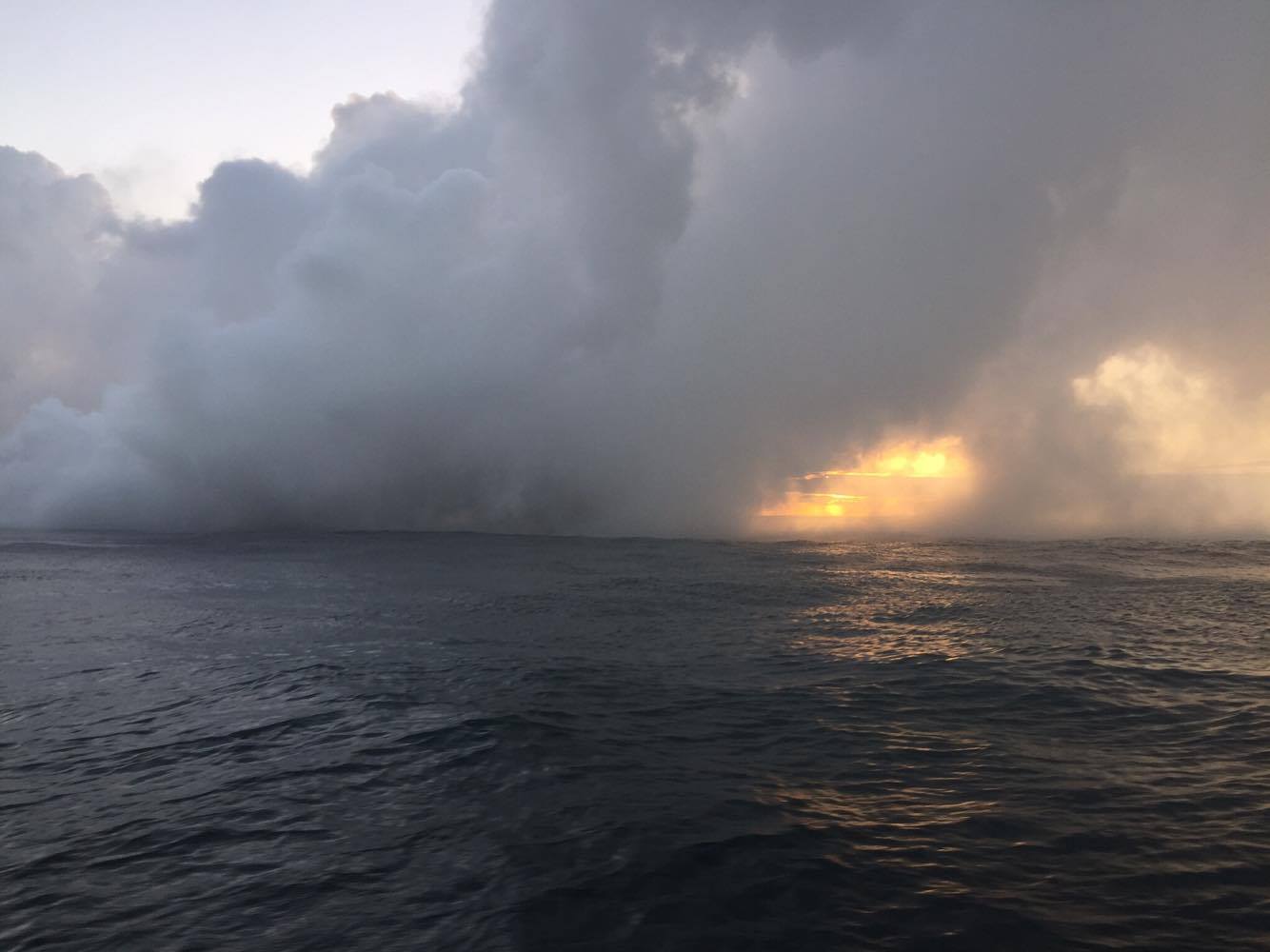
Kīlauea lava ocean entry, 10.13.16. PC: Robin Pilus.